Astronomical objects & observations
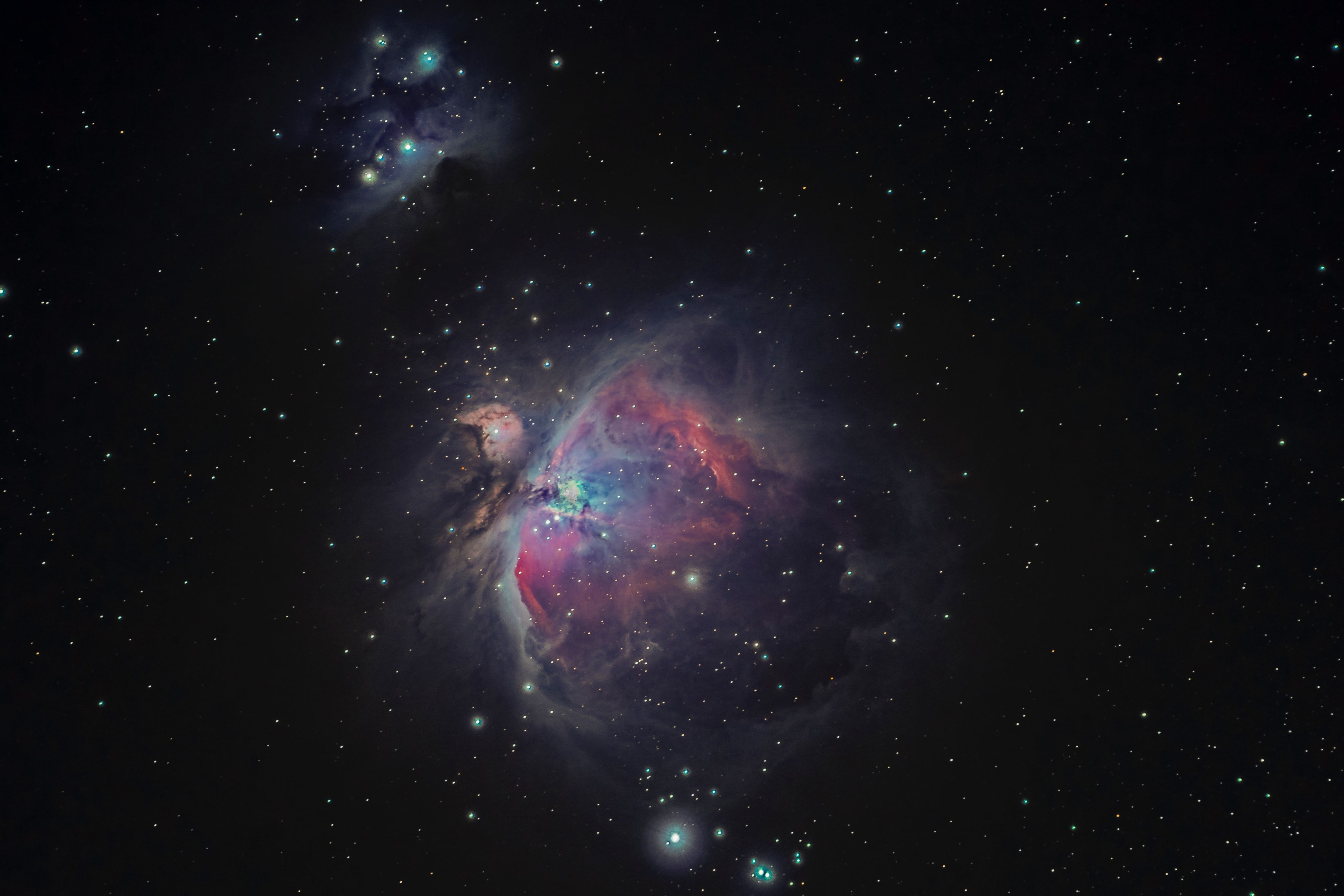
In 2017 Cathie became the first woman to receive the Eddington Medal, awarded by the Royal Astronomical Society for investigations of outstanding merit in theoretical astrophysics. In 2022 she became the first female director of the Institute of Astronomy at the University of Cambridge, and in May 2023 she was elected a Fellow of the Royal Society.
In this article, Cathie discusses how the use of theoretical models on how astronomical objects may behave allows us to make predictions over billions of years…
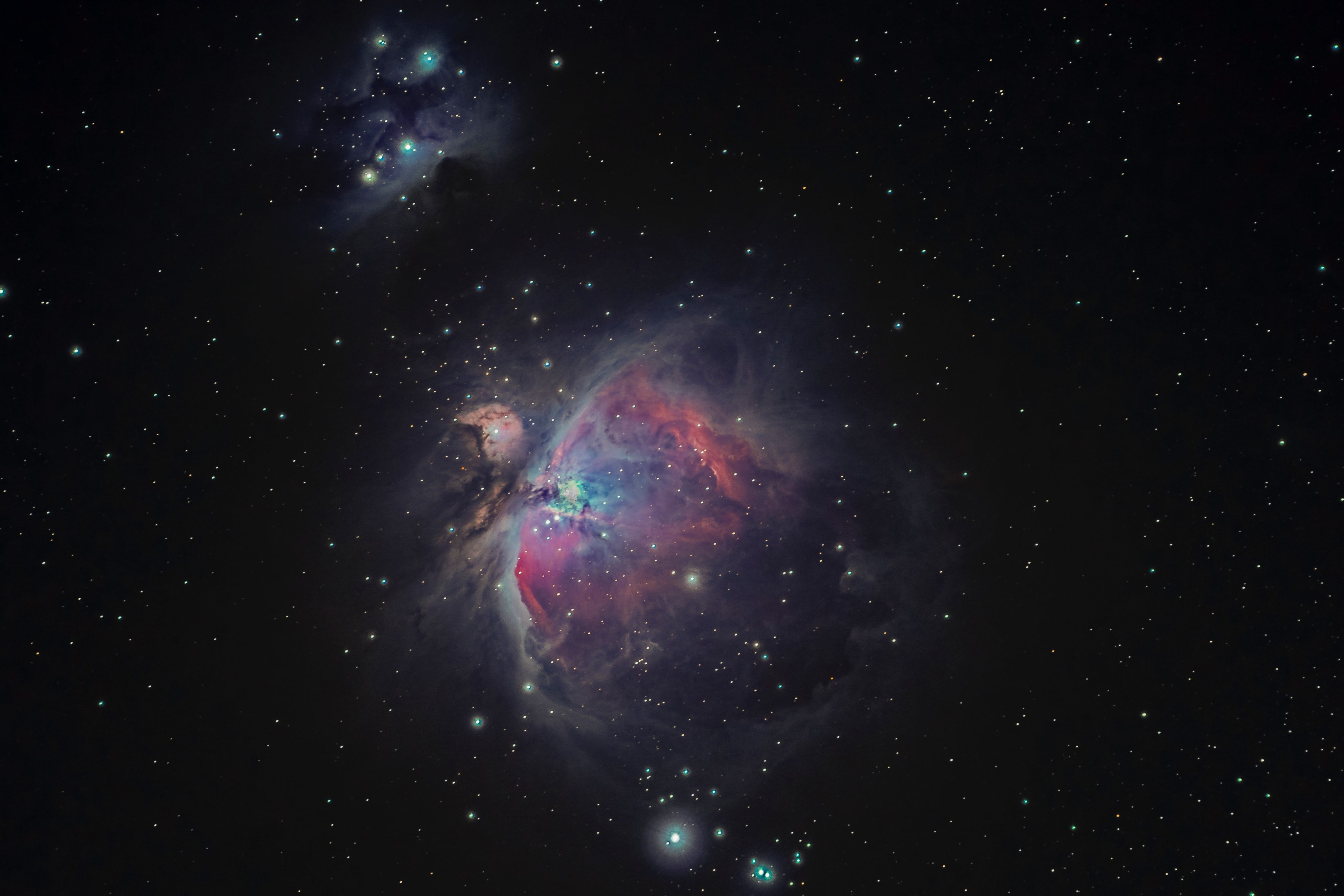
I am a theoretical astrophysicist so I spend my time trying to understand what observations are telling us about the workings of astronomical objects. While popular science often highlights the stunning images that come from exciting new facilities such as the James Webb Space Telescope, what is often not emphasized is how astronomers use images and spectra to select between contending models. This is where people like myself come in: I devise theoretical models which set out that if such and such a process is operative then this should produce a particular observational signature. Having a model is important because you can then go on to make predictions about aspects of the system that cannot be observed directly. In the case of astronomy this often involves being able to predict the behavior of systems over timescales (millions to billions of years) that are not amenable to observations.
My particular interest is in protoplanetary discs which are composed of dust and gas orbiting young stars. Such discs were first postulated by Kant and Laplace in the eighteenth century as being a precursor to the planetary system around our Sun. Indirect confirmation that discs were widespread around young stars was provided by infrared satellite data in the 1980s but high resolution imaging of such discs has only been possible in recent years thanks to the capabilities of the Atacama Large Millimetre Array (ALMA). A beautiful ALMA image of a protoplanetary disc was published by my group in 2018 (Figure 1). What was particularly exciting about this image was the set of dark rings which are interpreted as regions that have been cleared of dust by very young planets. This was one of the first demonstrations that planets are indeed apparently forming in discs. Identifying and characterizing these youngest planets and thereby helping to understand their formation is a major current effort worldwide.
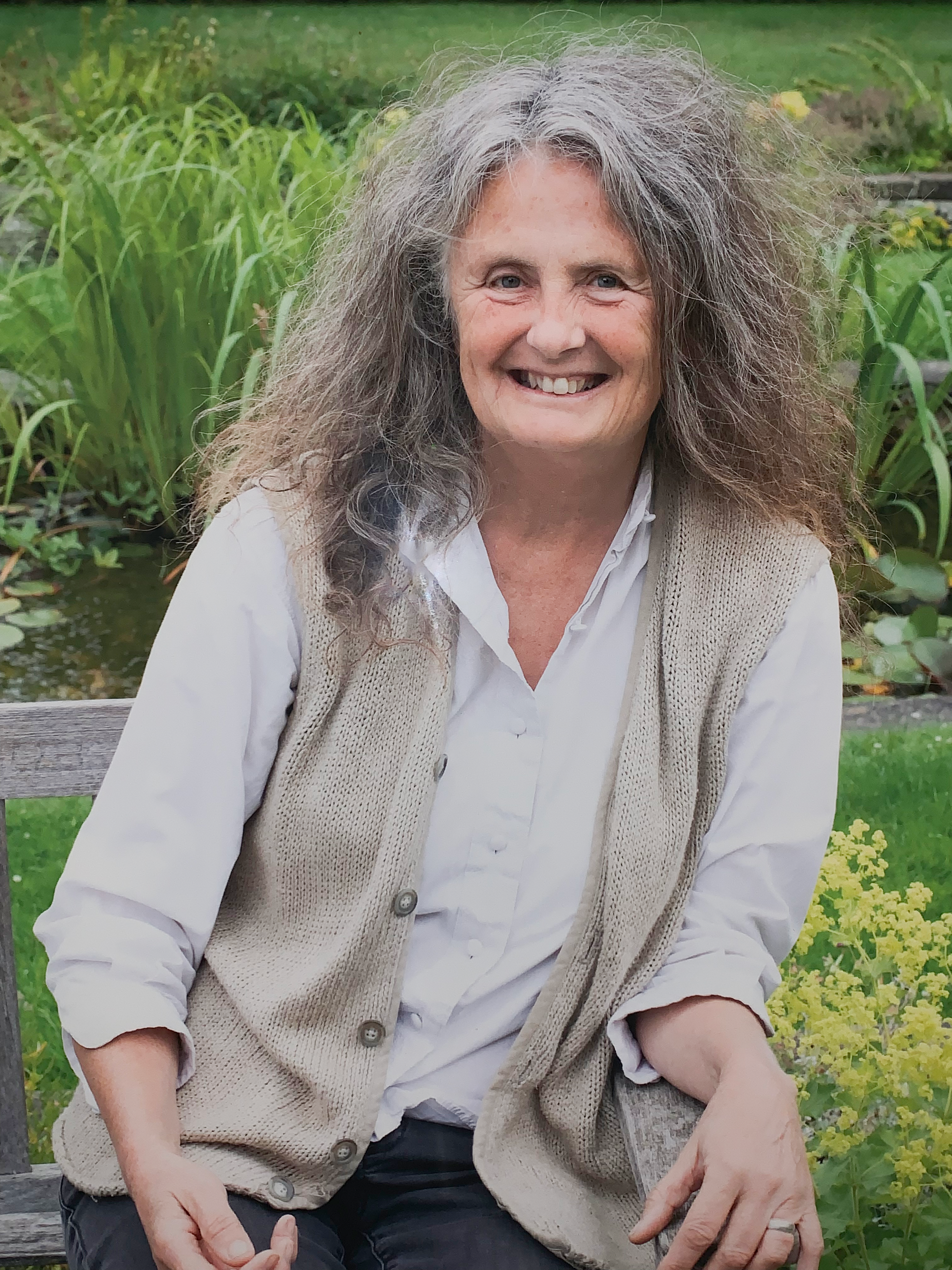
Professor Cathie Clarke
Professor Cathie Clarke
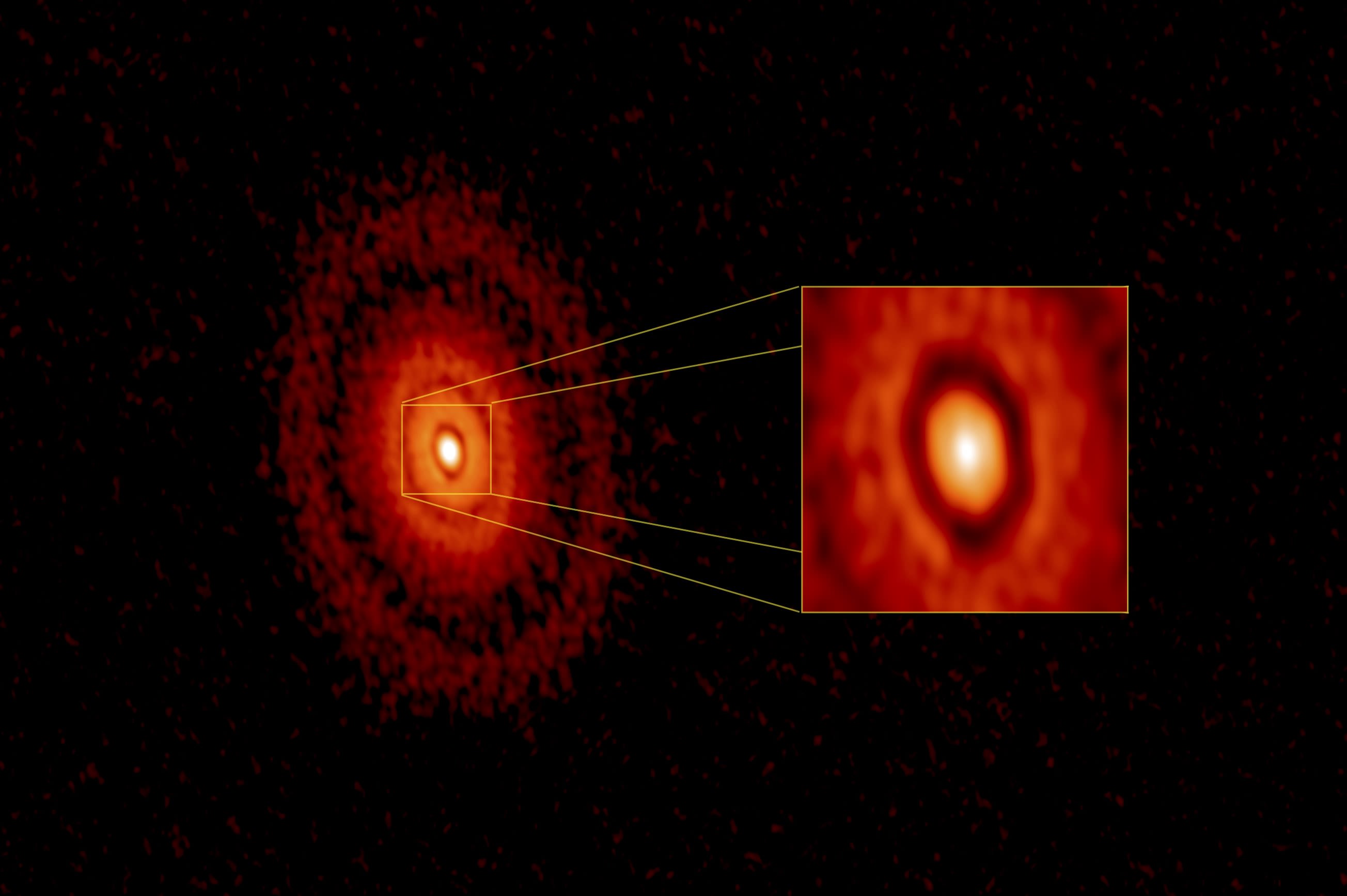
Figure 1
Figure 1
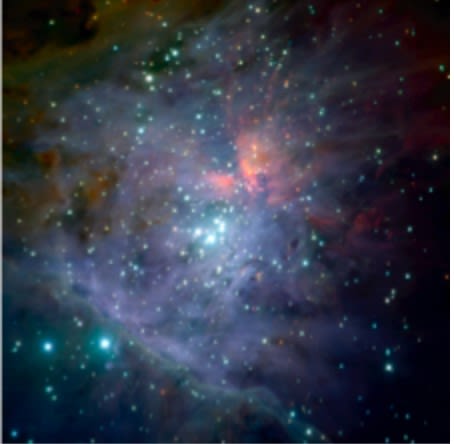
Figure 2
Figure 2
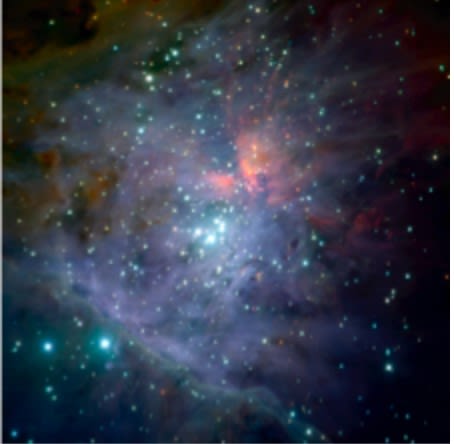
Figure 2
Figure 2
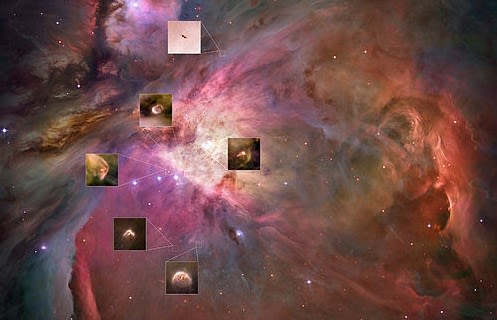
Figure 3
Figure 3
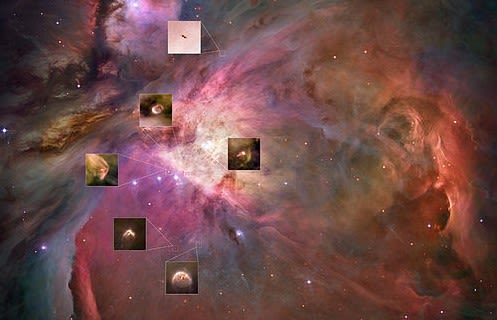
Figure 3
Figure 3
I am particularly interested in the processes that disrupt discs and terminate the process of planet formation. I sometimes describe this as the `environmental science of planet formation’ because it turns out that the chance that planets are able to form is very dependent on the local star forming environment in which the nascent protoplanetary disc is located. Our own Galaxy presents a great diversity of stellar birth environments, ranging from the relatively sparse star forming regions located near the Sun to denser and more populous regions such as the Orion Nebula Cluster (Figure 2) and even denser clusters such as the Arches Cluster located near the Galactic Centre. I have shown that the very high ultraviolet radiation fields generated by massive stars in these dense and populous environments has a major impact on the lifetime and hence planet forming capacity of discs: this is because such fields drive strong winds from discs through a process known as photoevaporation. We therefore need to be cautious about building a picture of planet formation using observations of the sparse environments that are near the Sun and recognize that these regions, whilst easiest to study, are probably giving us an unrepresentative picture of planet formation throughout the Galaxy.
How can one test predictions about the way that protoplanetary discs are boiled away in regions of high ultraviolet flux? We need to compare with observations such as the images in Figure 3: these sources in the Orion Nebula Cluster show characteristic tadpole shaped structures which contain an often invisible disc orbiting a low mass star. Their bright rims trace where ionizing radiation from neighbouring massive stars interacts with powerful winds blowing off the disc.
Recent research has unveiled the intricate dance of microbial life within us. Different regions of the Comparison of models and observations is however often limited by the computational expense of running large numbers of hydrodynamical models: for this reason it is always very welcome when it is possible to work out solutions with pen and paper mathematics. In this case large numbers of models can then be generated very easily. I was particularly pleased therefore to have come up with such an analytic model for disc winds a few years back. This was significantly more complex than the classic problem of a wind blowing from the surface of a spherical star where the flow trajectories are purely radial. The disc wind is more complicated because it is necessary to solve simultaneously for the morphology of the flow and the variation of quantities along streamlines.
As I write we are using these models to compare with new data on disc winds obtained with the James Webb Space Telescope in its first Cycle of operation.
It’s too early to say what we’re finding as there are many aspects of the data processing pipeline that have to be carefully checked. But for a theorist like myself, it is an exciting moment when you get to compare your theory with `real world’ observations. Fortunately we can look forward to much more data of this kind in the coming years….